Photosynthesis proteins include photoharvesting proteins, energy transfer proteins, reaction center proteins and photo protective proteins. For all these systems, energy dissipation is critical. A key mechanism of energy dissipation is through the energy transfer to the vibrations within the macromolecules. These vibrations are in the terahertz frequency range. In addition, for some photo protective proteins, large scale conformational change occurs as they transition from the resting state to the photo protective state, and this transition may be facilitated by the directed sampling of atomic displacements biased by the protein structure and intramolecular interactions. We are using ATM to characterize the vibrations of photosynthesis proteins to examine the overlap of the energies with energy differences for electronic states between the chromophores, as well as the structural motions associated with the vibrations that enable efficient conformational transitions
|
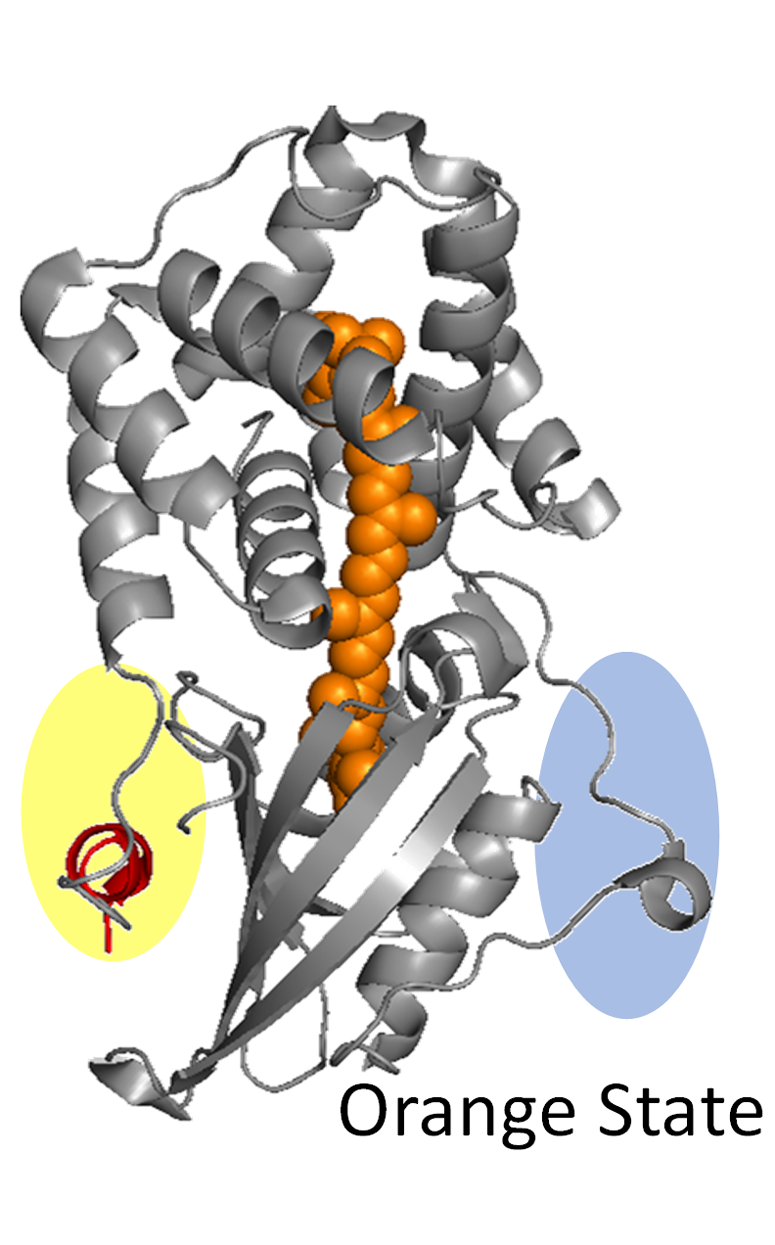 |